Research
Living systems are able to execute complex biological functions such as growth, organization, and learning. These functions are sustained by continuous consumption of free energy, which drives living systems out of equilibrium. My research aims to use the tools of nonequilibrium statistical physics to understand the mechanisms underpinning important biological functions.
- Spatiotemporal organization in living systems: Pattern formation and intracellular phase separation
- Information processing in a single cell: Bacterial chemotaxis signaling
- Nonequilibrium thermodynamics of biological functions: Energy cost and trade-offs
- Statistical physics models of neural activity
Spatiotemporal organization in living systems: Pattern formation and intracellular phase separation
How do cells organize their internal structures? How do they ensure that molecules appear at the right place and time? Through close collaboration with experimentalists, we are constructing continuum models to interrogate how spatiotemporal order emerges from microscopic interactions, in biological systems ranging from lipid membranes to chromosome segregation in bacteria and nucleolar architecture in human cells.
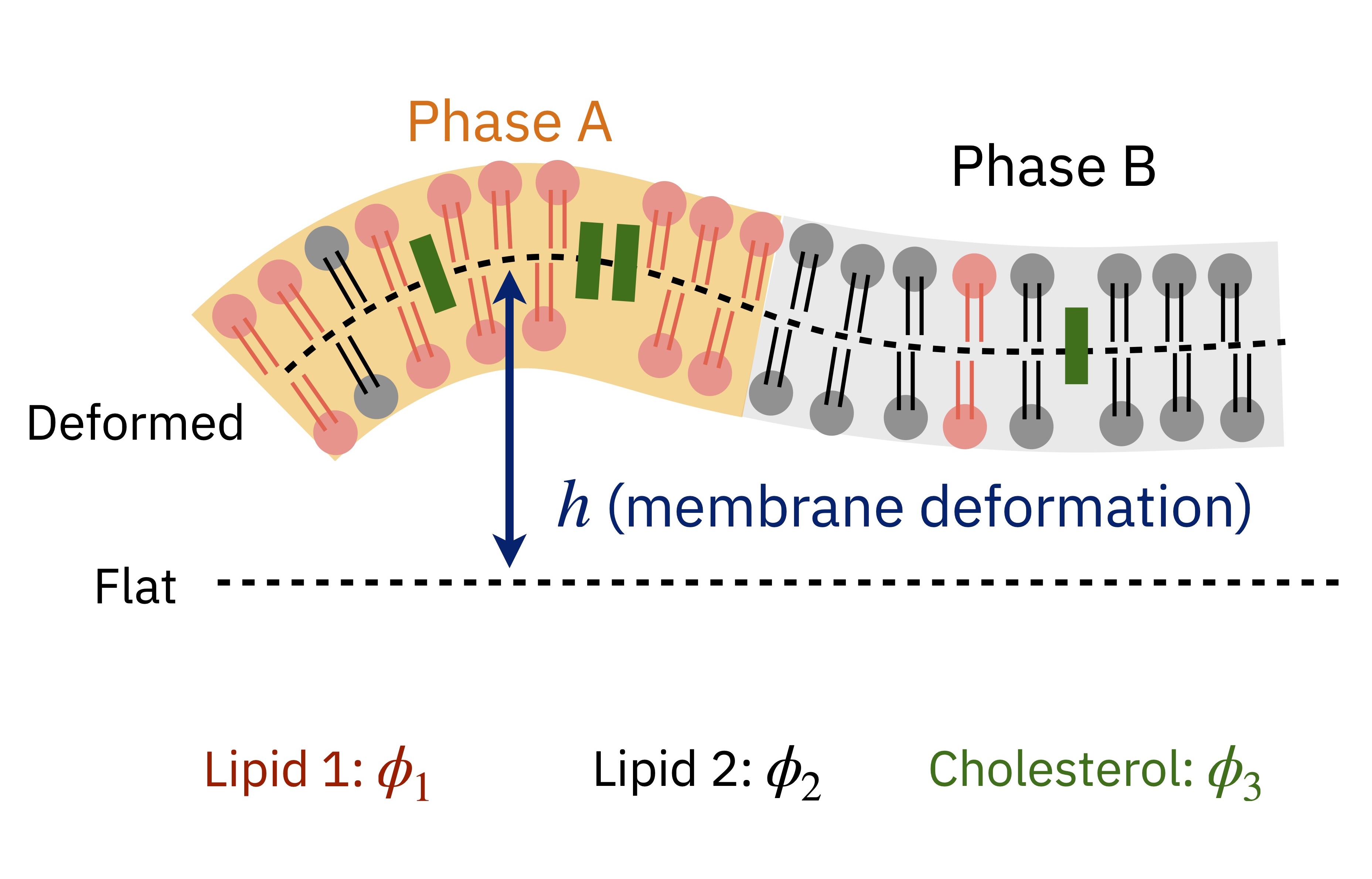
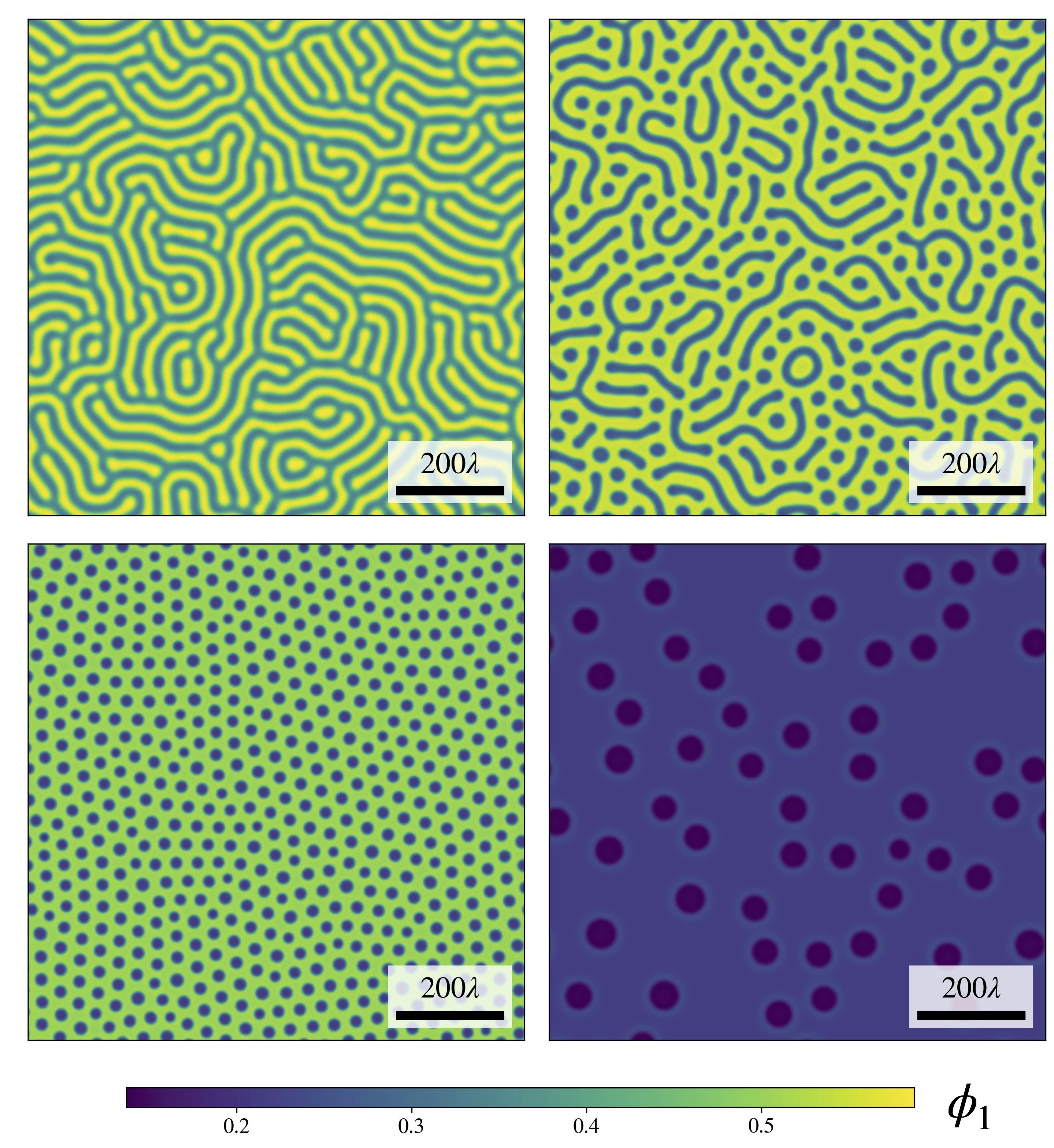
Publications
- A. Papagiannakis, Q. Yu, S. K. Govers, W-H Lin, N. S. Wingreen, C. Jacobs-Wagner, Nonequilibrium polysome dynamics promote chromosome segregation and its coupling to cell growth in Escherichia coli, eLife 14, RP104276 (2025). [bioRxiv link] [eLife link]
- X. Ouyang, S. Sutradhar, O. Trottier, S. Shree, Q. Yu, Y. Tu, J. Howard, Neurons exploit stochastic growth to rapidly and economically build dense radially oriented dendritic arbors, bioRxiv 2025.02.24.639873 (2025). [bioRxiv link]
- S. A. Quinodoz$^{=}$, L. Jiang$^{=}$, A. Abu-Alfa, T. Comi, H. Zhao, Q. Yu, …, A. Košmrlj, D. L. Lafontaine, S. Klinge, C. P. Brangwynne, Mapping and engineering RNA-controlled architecture of the multiphase nucleolus, bioRxiv 2024.09.28.615444 (2024) [bioRxiv link]
- Q. Yu and A. Košmrlj, Pattern formation of phase-separated lipid domains in bilayer membranes, arXiv 2309.05160 (2023). [arXiv link] [PDF].
Information processing in a single cell: Bacterial chemotaxis signaling
How do bacteria navigate in complex environments? This simple question has been studied for decades in the model bacterium Escherichia coli to reveal general principles of cellular information processing. By sensing their environment, bacteria can swim toward nutrients and away from toxins. This chemotaxis behavior is achieved with a simple biochemical network: first, receptors on the cell surface bind to chemical attractants; this measurement is then propagated to activate messenger proteins within the cell, which in turn control the flagella, the motors that propel the bacteria forward or change its heading.
Nevertheless, recent experiments and theory are beginning to show a new paradigm that highlights the importance of nonequilibrium cooperative interactions in signaling. Our recent works reveal two signatures of irreversible dynamics in chemotaxis signaling. First, receptor methylation shifts the kinase response curve by orders of magnitude in ligand concentration while incurring a much smaller change in the ligand binding curve. Second, the chemosensory array exhibits spontaneous asymmetric switching between fully active and fully inactive states. We constructed a nonequilibrium allosteric model (Hathcock$^{=}$, Yu$^{=}$, et al., PNAS 2023) and a nonequilibrium lattice model (Hathcock$^{=}$, Yu$^{=}$, Tu, Nature Communications 2024) to explain these observations. Our results highlight the role of energy dissipation in enhancing the speed, sensitivity, and robustness of chemotaxis signaling, and provide a new perspective on cooperative sensing by large protein complexes.
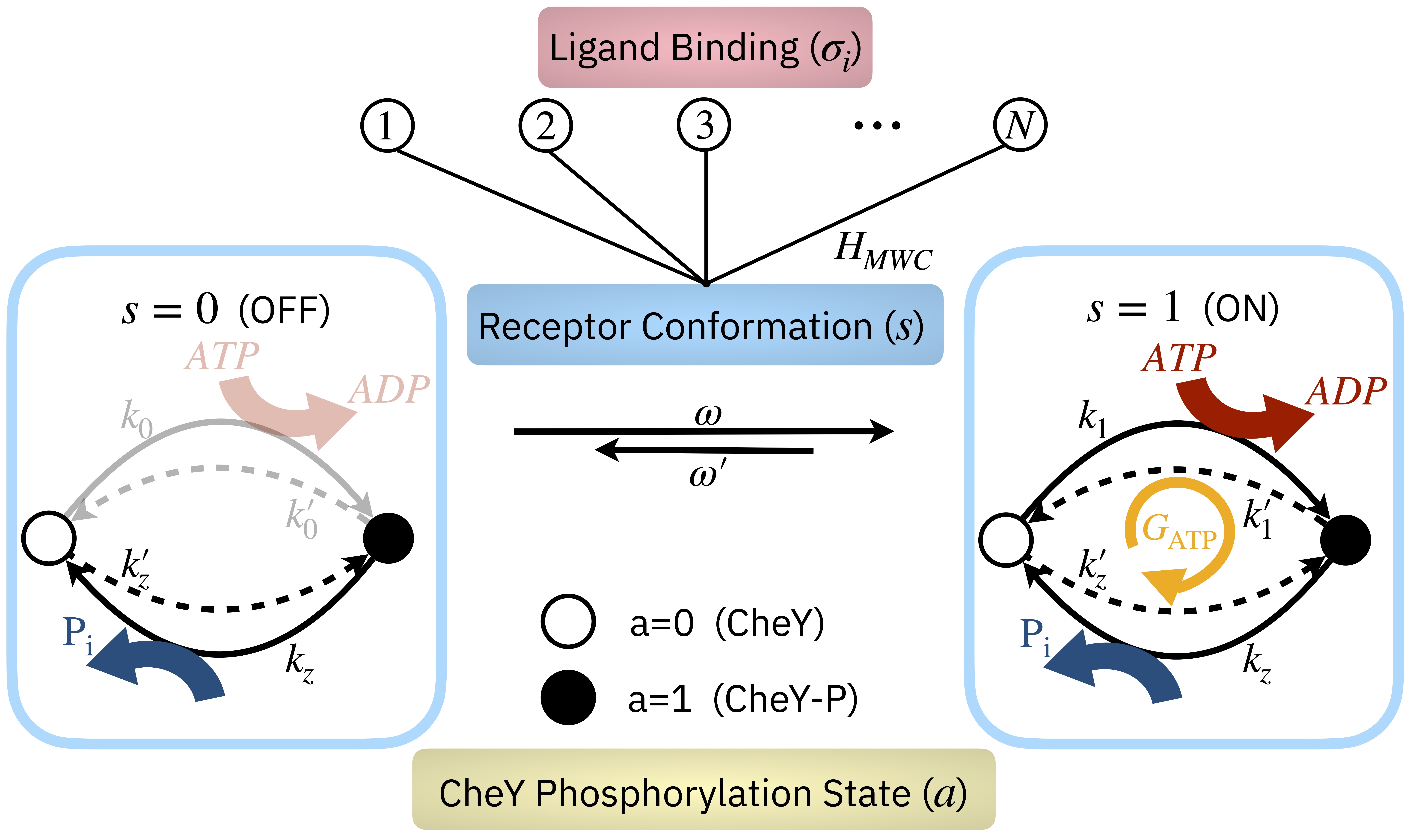
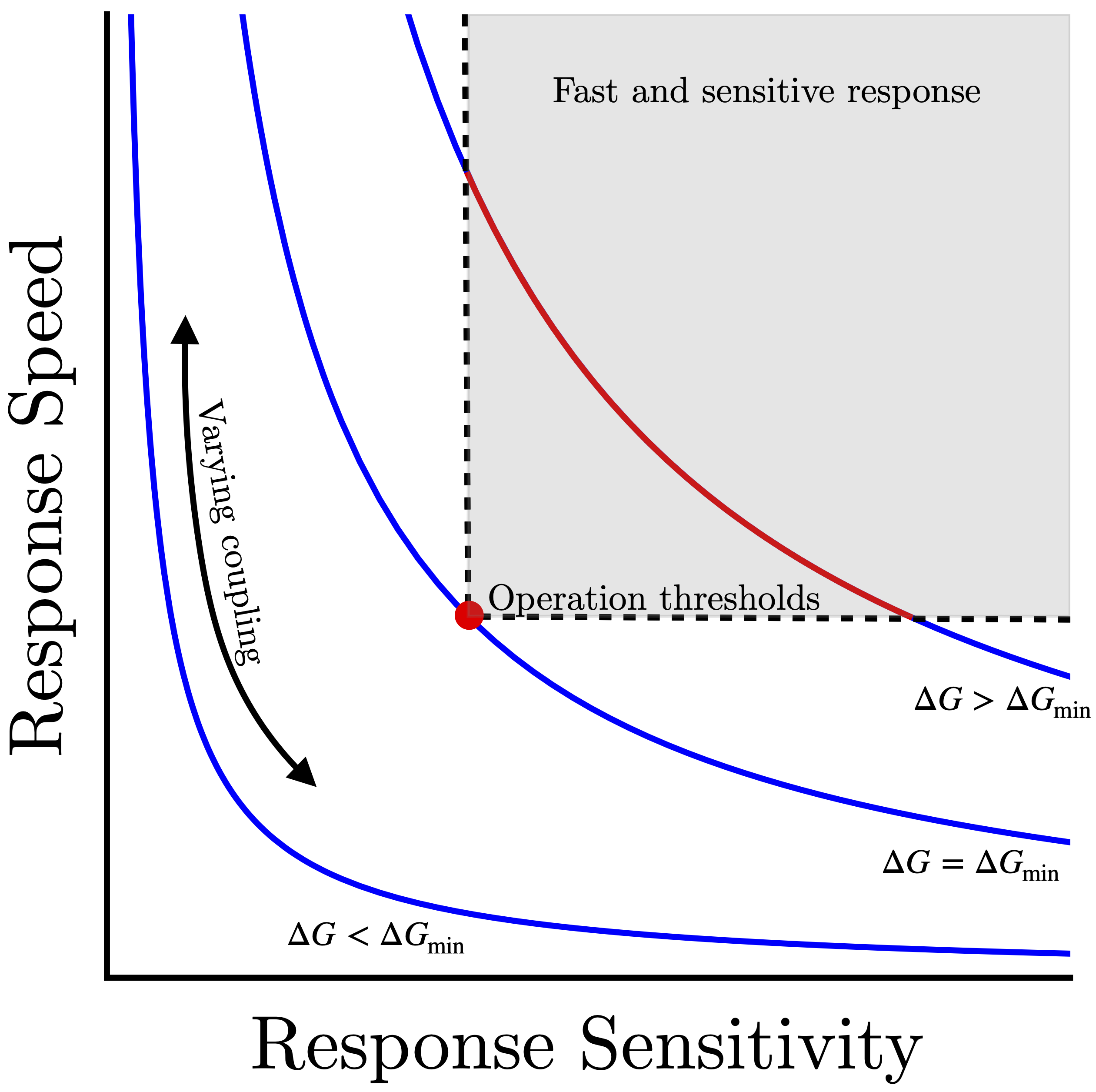
Publications
- D. Hathcock$^{=}$, Q. Yu$^{=}$, and Y. Tu, Time-reversal symmetry breaking in the chemosensory array: asymmetric switching and dissipation-enhanced sensing, Nature Communications, 15, 8892 (2024). [Journal link] [PDF].
- D. Hathcock$^{=}$, Q. Yu$^{=}$, B. Mello, D. N. Amin, G. L. Hazelbauer, and Y. Tu, A nonequilibrium allosteric model for receptor-kinase complexes: The role of energy dissipation in chemotaxis signaling, Proceedings of the National Academy of Sciences, 120, e2303115120 (2023). [Journal link] [PDF].
Nonequilibrium thermodynamics of biological functions: Energy cost and trade-offs
Living systems are driven far from equilibrium. Energy dissipation is crucial for a wide range of biological functions such as adaptation, error correction, environmental sensing, and collective motion. Using the tools of stochastic thermodynamics, we are studying how energy dissipation places fundamental limits on the performance of biological systems, such as flocking (Yu and Tu, PRL 2022) and kinetic proofreading (Yu et al., J. R. Soc. Interface 2022). We are also interested in extending the concepts of coarse-graining and the renormalization group from equilibrium to nonequilibrium systems (Yu et al., PRL 2021).
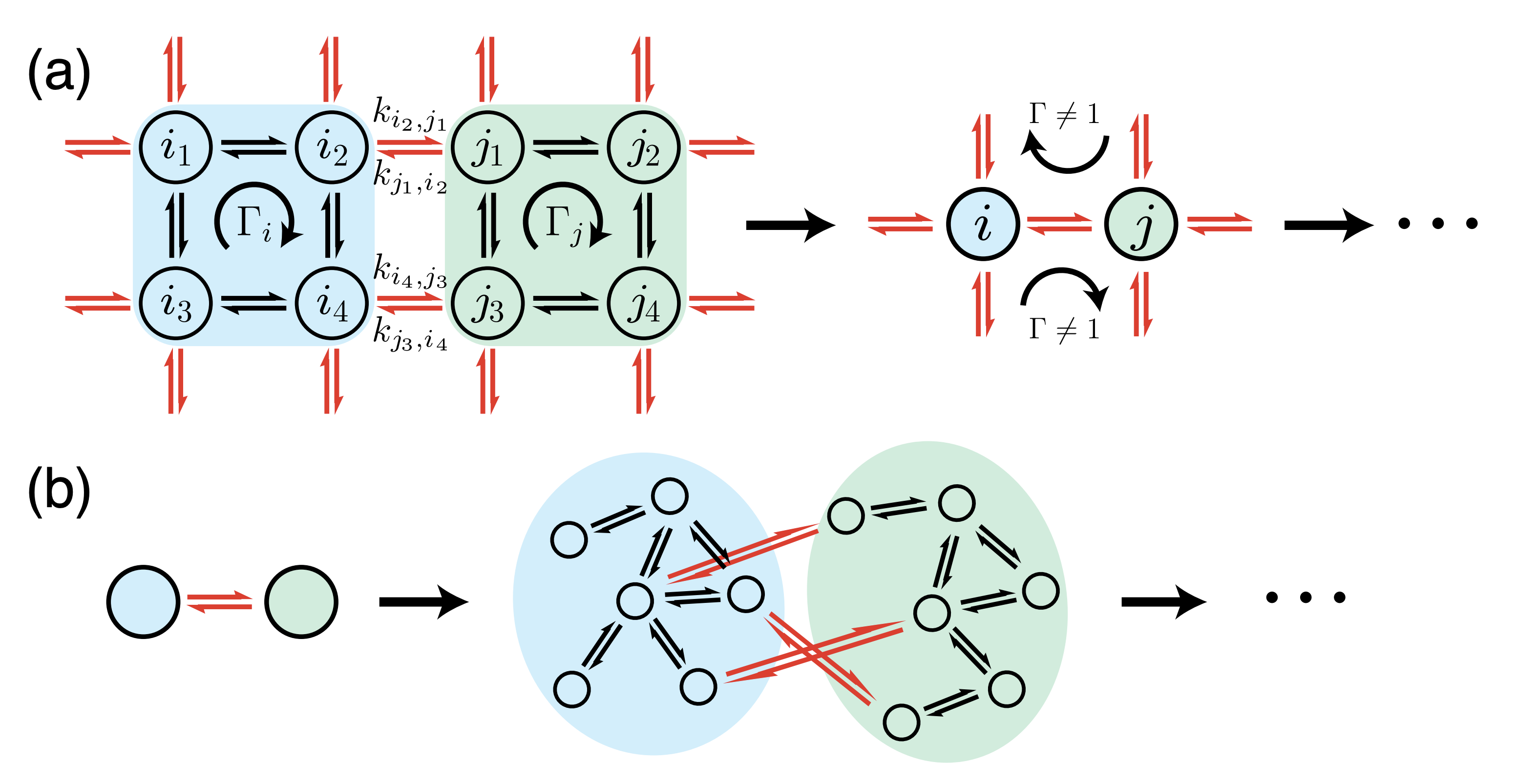
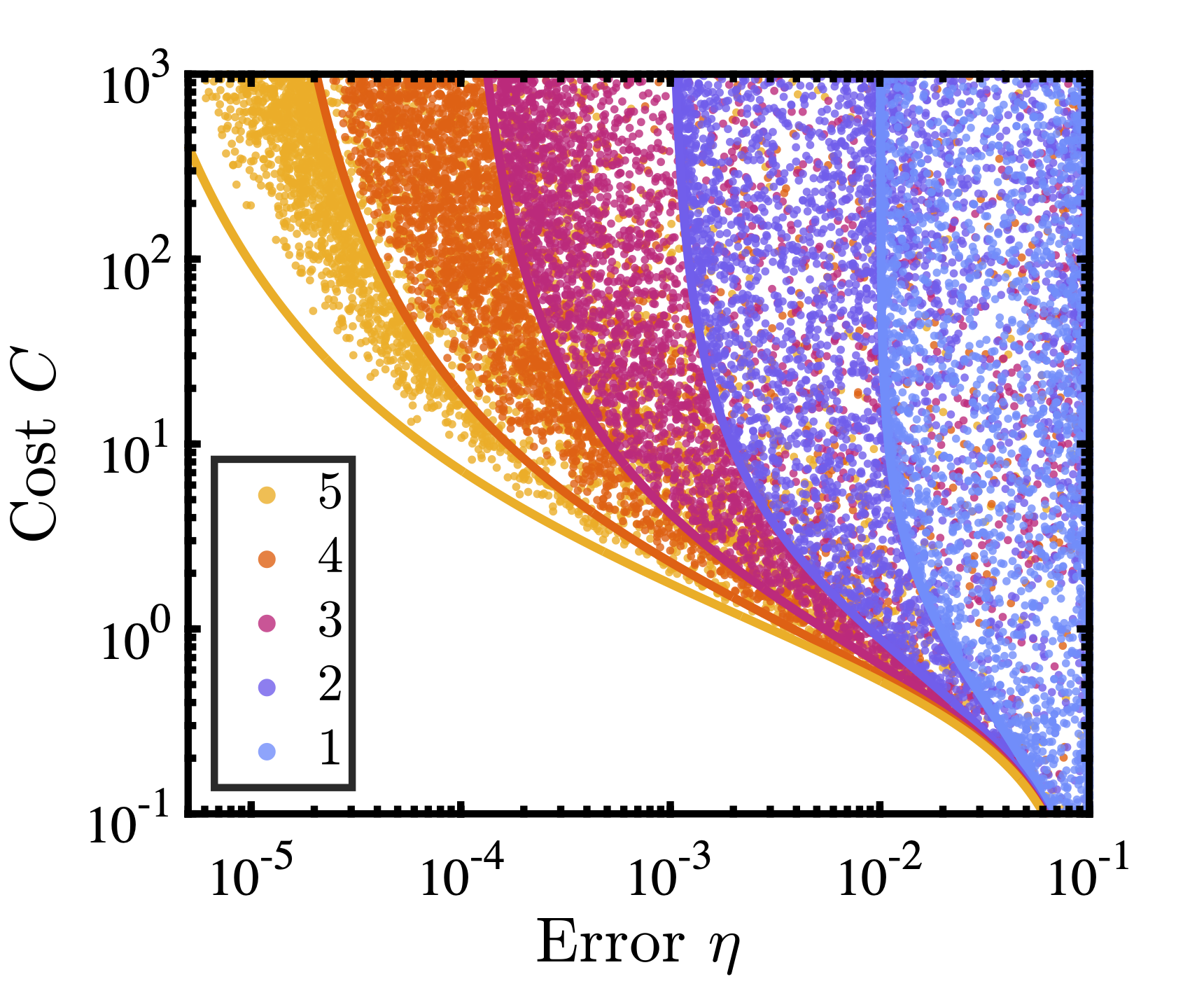
Publications
- Q. Yu$^{=}$ and P. E. Harunari$^{=}$, Dissipation at limited resolutions: Power law and detection of hidden dissipative scales, Journal of Statistical Mechanics: Theory and Experiment, 2024, 103201 (2024). [Journal link] [PDF].
- Q. Yu and Y. Tu, Energy cost for flocking of active spins: The Cusped Dissipation Maximum at the Flocking Transition, Physical Review Letters, 129, 278001 (2022). [Journal link] [PDF].
- Q. Yu and Y. Tu, State-space renormalization group theory of nonequilibrium reaction networks: Exact solutions for hypercubic lattices in arbitrary dimensions, Physical Review E, 105, 044140 (2022). [Journal link] [PDF].
- Q. Yu, A. B. Kolomeisky, and O. A. Igoshin, The energy cost and optimal design of networks for biological discrimination, Journal of the Royal Society Interface, 19, 20210883 (2022). [Journal link] [PDF].
- Q. Yu, D. Zhang, and Y. Tu, Inverse Power Law Scaling of Energy Dissipation Rate in Nonequilibrium Reaction Networks, Physical Review Letters, 126, 080601 (2021). [Journal link] [PDF] .
- Q. Yu, J. D. Mallory, A. B. Kolomeisky, J. Ling, and O. A. Igoshin, Trade-Offs between Speed, Accuracy, and Dissipation in tRNA$^{Ile}$ Aminoacylation, The Journal of Physical Chemistry Letters, 11, 4001-4007 (2020). [Journal link] [PDF]
Statistical physics models of neural activity
Publications
- C. W. Lynn, Q. Yu, R. Pang, W. Bialek, and S. E. Palmer, Exactly solvable statistical physics models for large neuronal populations, Physical Review Research, in press (2025). [arXiv link]
- C. W. Lynn, Q. Yu, R. Pang, S. E. Palmer, and W. Bialek, Exact minimax entropy models of large-scale neuronal activity, Physical Review E, in press (2025). [arXiv link]